Research
Our group works in the interdisciplinary area bridging GPU-based quantum chemistry, chemical kinetics, fluid mechanics, and gas dynamics, with the aid of machine learning and data-driven methods. We develop multiscale modeling approaches for reacting flows and energy materials to advance aerospace sustainability, and high-speed propulsion, and clean energy transition.
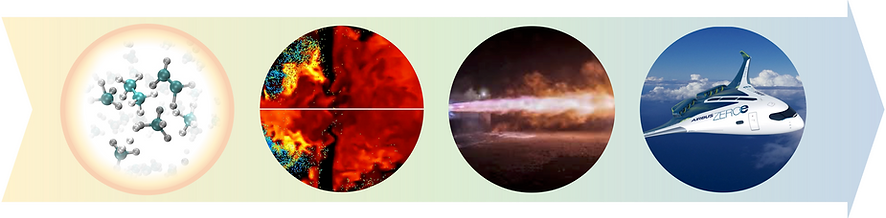
Research Directions
Aerospace sustainability
As the global demand for air travel continues to rise, the aerospace carbon footprint is increasingly concerning. To mitigate green house gas emissions, it is highly desirable for the efficient certification and mass production of sustainable aviation fuels (SAF), a bio-derived, carbon-neutral jet fuels. Our group develop efficient modern computational tools to explore the SAF design pathways, and model the combustion and emission behavior. This will enable rational SAF design, and efficient SAF testing for both current and future air-breathing propulsion systems.
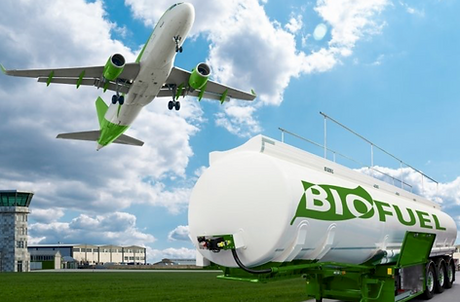
Image source: DOE

Image source: NASA
High-speed reacting flow
Understanding high-speed reacting flow physics is critical for the design of next-generation high-power, high-speed aerospace vehicles. Phenomena such as thermal nonequilibrium, ultrafast turbulent mixing, and shock-chemistry interactions are still not well understood. Our group aims to leverage quantum chemistry, machine learning, and gas dynamics to establish a multiscale approach for high-speed reacting flow. We are particularly interested in nonequilibrium aerothermochemistry, shock-chemistry interaction, and low-temperature plasma problems.
Sustainable energy materials
Recent advances in optical physics and synthetic chemistry enabled the design of materials that can undergo controlled shape changes under light activation. Challenges remain at the theoretical level to explain, predict, and help to design these materials for sustainable energy conversion. Our group works at the intersection of excited-state quantum chemistry, force-induced molecular mechanics, and continuum mechanics to investigate material chemistry at the molecular scale, and mechanical deformation at the continuum scale. Applications include light-harvesting materials, stress-responsive polymers, and batteries.
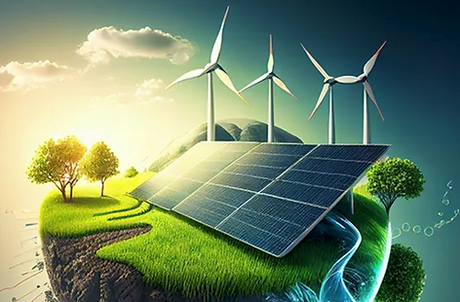
Image source: green.org
Research Approach
Our group tackles the challenges to bridge the scales in the modeling of reacting flows and solids. We develop computational tools to leverage GPU-accelerated quantum chemistry, chemical kinetics, fluid mechanics, and gas dynamics, with the aid of machine learning and data-driven methods. ​
-
Below is an example of multiscale reacting flow modeling. Our computation approach will span across different time and length scales, featuring interactions between the microscopic, molecular scale (e.g., fast chemistry and thermal nonequilibrium) and the macroscopic, flow scale (e.g., shock, boundary layer, and turbulent flow).

-
An example on applying fast (GPU-based) quantum chemistry modeling for reacting flow simulation is highlighted in one of Rui's past work. (R. Xu, et al., Chem. Sci., 14, 7447-7464, 2023.)